Pain is a strange sensation unlike any other we experience.
While most of our senses have a clear, specific stimulus they’re responding to, pain doesn’t play by those rules.
Vision is all about light, picking up on a certain part of the electromagnetic spectrum. Hearing is all about sound waves.
But pain? Pain doesn’t have a single special stimulus. It’s often a response to tissue damage or the threat of tissue damage, but that’s not always the case. Sometimes, we feel pain when there is no threat or damage.
We know pain — it’s that raw, primal feeling that can turn the strongest among us into a curse-word jackpot machine.
And yet, despite its familiarity, pain remains one of the more puzzling phenomena in neuroscience. It turns out that explaining what’s going on in the brain during these seemingly simple events is not so simple.
In last week’s article, we explored Gertler’s thought experiment on disembodied pain. We discussed her view that pain has no hidden essence — that pain is a subjective experience fully accessible through introspection. This week, as we explore the neuroscience of pain, we might want to ask:
Can neuroscience reveal anything about the nature of pain that we can’t get through introspection alone?
In neuroscience, we think of pain as a combination of sensation with emotion and cognition. These three aspects of pain follow different pathways in the brain.
The painfulness pathway
The suffering pathway, and
The pain suppression pathway
This week, let’s review these three pain pathways and explore how they work together to create our complex experience of pain.
But first, let’s think about…
Why We Need Pain
Pain is something we all want to avoid. But here’s the paradox: pain is actually a good thing.
Evolutionarily speaking, pain is our body’s built-in alarm system. It warns us to stop harmful activities, seek treatment, protect ourselves from further damage, or rest to heal.
The benefits of pain are probably best illustrated by people who don’t feel it. Some folks are born with a condition called congenital insensitivity to pain. Which, on first reflection, might sound great, but it’s an awful condition to have.
Most cases of congenital insensitivity to pain result from mutations in genes that are crucial for pain sensation. These mutations can affect how the neurons involved in pain develop, making those neurons function incorrectly or, in some cases, die off entirely.
One of the more famous cases of congenital insensitivity to pain is that of Miss C, a student who attended McGill University in Montreal. She was a normal, intelligent woman in every respect, except she never felt pain.
When tested, she had no response to electric shock, hot water, or ice baths. If you’ve ever tried an ice bath, you know your body has a strong opinion about it. Blood pressure rises, heart rate increases, and breathing changes in response to the sudden chill. But Miss C? Nothing.
Interestingly, she never sneezed or coughed, and she never blinked her eyes when they needed protection. As you can imagine, this lack of response to potentially harmful situations was a big problem. As a child, she bit off the tip of her tongue; she often burned herself and broke several bones without realising it.
We may have had moments when we wished pain didn’t exist, but not feeling pain is a terrible condition to have. Someone who doesn’t feel pain never gets the signals to change their body position. They rarely roll over during sleep or shift when sitting and standing. When people don’t move, their bones and joints face constant, unrelieved pressure. Without the natural shifts and adjustments that come with movement, these bones and joints gradually deteriorate.
People who suffer from congenital insensitivity to pain typically die very young from complications. In the case of Miss C, she sadly died at the age of 29 from infections of her skin and bone trauma.
1: The Painfulness Pathway
Imagine you’re cooking dinner. In a moment of distraction, you forget to grab the oven mitt, and you grasp the handle of a hot pan with your bare hand. The pain hits you instantly — sharp and intense. You quickly pull your hand away, but the burning sensation lingers. Over the next few hours, the pain changes: the acute sting fades, replaced by a throbbing ache. How can a single event produce such a varied pain experience?
Pain sensation is closely related to touch sensation. Both pain and touch sensations rely on receptors. We find receptors at the end of certain types of neurons — receptor neurons. These receptor neurons detect changes in the environment and your body. We have receptor neurons almost everywhere in the body, including our joints, internal organs, and tissues.
In the skin, these receptor neurons pick up changes on the skin’s surface. So when you touch that hot pan with your bare hand, receptors in your hand detect the extreme temperature and send a signal up your arm to your spinal cord. At the spinal cord, these neurons synapse with other neurons that send the signal to the brain.
The receptor neurons that detect changes associated with pain are called nociceptor neurons. Nociceptor neurons are designed to identify damage and potentially harmful stimuli. We have specialised nociceptor neurons for detecting extreme temperatures, physical force or pressure, and certain chemicals.
When you first touch a hot pan, the extreme heat activates thermal nociceptor receptors in your skin (the ones that respond to extreme temperatures).
The axons of most thermal nociceptors are covered with a fatty substance called myelin. Myelin helps the neuron send its signal faster. This is why you feel almost immediate pain after touching something hot. Nociceptor neurons that are myelinated are called A-delta fibers.
It’s important to note that while nociceptors are often referred to as pain neurons, this is a bit of a misnomer. Nociceptors don’t create the sensation of pain all by themselves. Instead, they’re specialised detectors for potentially harmful stimuli. The actual experience of pain involves the brain and other contextual information.
Most signals from myelinated A-delta fibres travel to the brain via the painfulness pathway. The painfulness pathway helps determine how intense the pain is, where it hurts, and what type of pain it is.
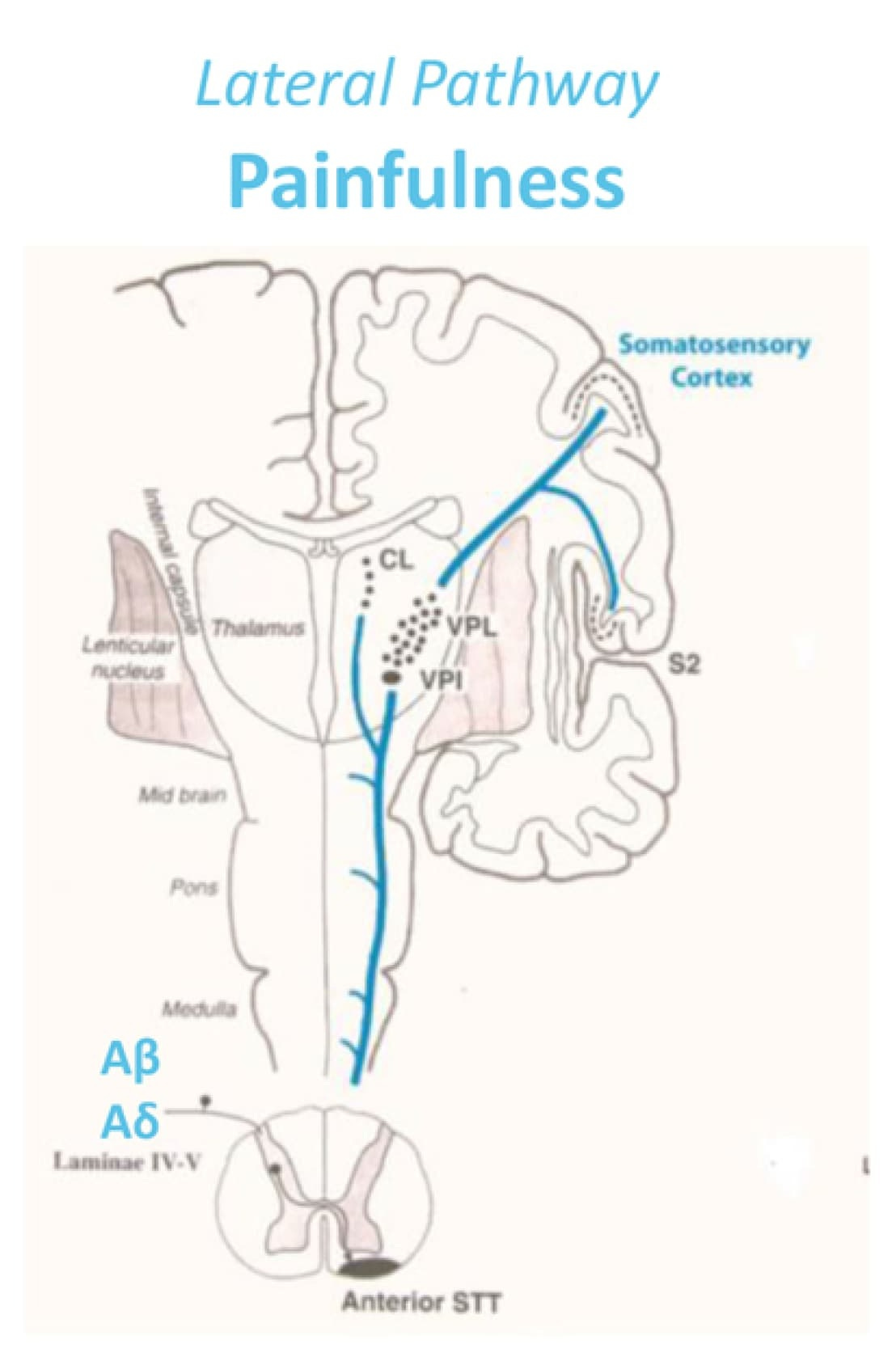
Although many areas are involved in the painfulness pathway, it is most known to involve the somatosensory cortex (purple in the image below).
You may recall that the somatosensory cortex contains a map of the body, with different areas representing different body parts. This map is mirrored in both the left and right hemispheres of the brain, with each hemisphere processing sensory information from the opposite side of the body. So, a touch on your left hand will be processed by the right somatosensory cortex, and vice versa.
We know the somatosensory cortex is involved in the sensation of pain because when people report pain in a certain body part, the corresponding area of the somatosensory cortex will be active.
But interestingly, the somatosensory cortex doesn’t seem sufficient, or maybe even necessary, for pain.
Patients who have had one hemisphere of their brain removed (a procedure sometimes done to treat severe epilepsy) can still feel pain on both sides of their body.
And if we directly stimulate the somatosensory cortex, the person might feel a sensation, but that sensation is typically not painful. Instead, they might experience tingling, pressure, or other non-painful sensations.
These findings tell us something important: while the somatosensory cortex is involved in pain, it’s not solely responsible for the experience of pain.
So, what else is going on in the brain during pain?
2: The Suffering Pathway
Like the painfulness pathway, the suffering pathway receives signals from inside the body and the skin. While this pathway does receive signals from A-delta fibres, most of its signals come from a different type of nociceptor neuron — C-fibers. C-fibers are unmyelinated receptor neurons. Without the myelin, signals from C-fibers are slower than signals from A-delta fibres.
You may have heard of C-fibers before. They are often described as the pain neurons. But we actually have two types of C-fibers — low and high threshold C-fibers. The low threshold C-fibers respond to gentle inputs like light touch and are typically associated with pleasure. High-threshold C-fibers respond to extremes and are typically associated with pain.
The suffering pathway includes areas like the anterior cingulate cortex (ACC), which processes the emotional aspect of pain. The emotional component of pain often lasts longer than the immediate sensory experience, which is why the unpleasantness can linger even after the sharp sensation has faded.
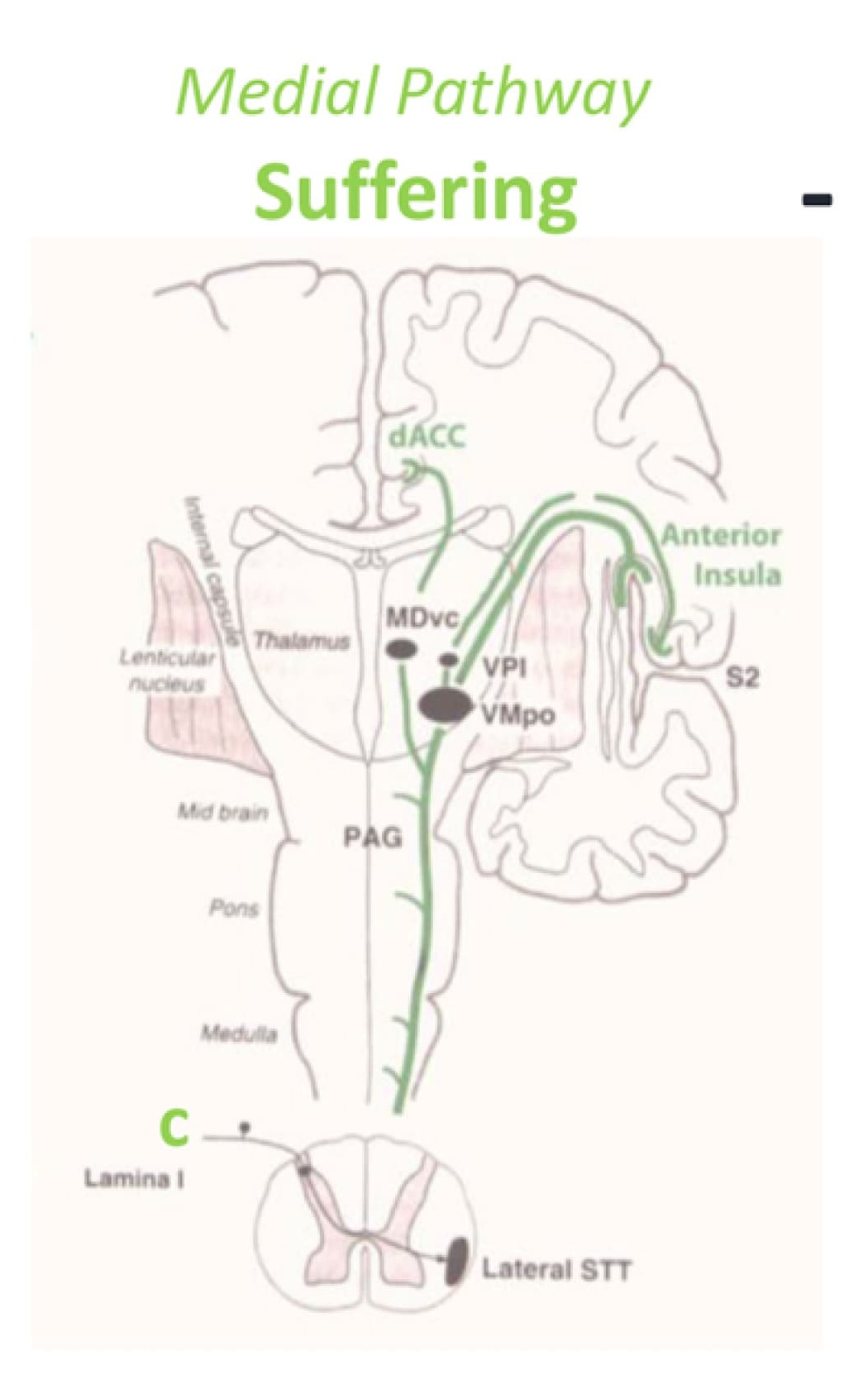
Some people with severe epilepsy or intractable pain have their ACC removed. Interestingly, these individuals still feel the sensory aspect of pain — they can identify where it hurts and describe its intensity and the physical sensation. But they often report that the pain no longer bothers them. In other words, they feel the pain, but they don’t suffer from it.
This striking dissociation between the sensory and emotional aspects of pain has also been shown under hypnotic suggestion. That is, someone might be hypnotised to feel the sensory aspects of pain without the accompanying distress. While under such hypnotic conditions, brain imaging showed changes that corresponded with their reported experiences.
These findings align with an influential theory — the Gate Control Theory of Pain. This theory suggests that pain signals can be modulated or gated.
This gating effect explains various pain phenomena. For instance, acute stress or arousal (like during an emergency or for soldiers in battle) can temporarily reduce pain sensation — the gates close, blocking pain signals. However, chronic stress often has the opposite effect. Prolonged stress can keep these gates open, amplifying pain signals and potentially contributing to conditions like chronic pain or fibromyalgia.
3: The Pain Suppression Pathway
While the painfulness pathway and the suffering pathway bring pain signals to the brain, the pain suppression pathway works top-down. This pathway allows the brain to modulate incoming pain signals based on factors like attention, expectation, and context.
One of the strange things about pain is that the same painful event doesn’t always seem to result in the same level of pain. While some people will barely notice a painful event, others might find the same event unbearable.
Even within the same person, pain perception can fluctuate. Some days, the gym feels bearable, maybe even enjoyable, and other days, the same routine is misery.
We see the pain suppression pathway at work when we consider how context can affect pain perception.
The power of context in shaping our pain experience is remarkable. For instance, a person might find a massage pleasurable and relaxing in a spa setting but experience discomfort or pain from the same pressure level applied in a medical setting.
Context in terms of cultural background and personal experiences also plays a significant role in pain perception. For instance, some religious rituals involve practices that would normally be considered excruciating but, when experienced as part of a ceremony or ritual, are not felt as pain. Many appear to be experiencing the entirely opposite state — one of extreme happiness.
The pain suppression pathway is also thought to be responsible for expectation-based pain modulation, such as placebo effects. Taking a placebo — a drug or procedure that has no pharmacological effects — can not only result in the placebo taker reporting less pain, but brain scans show less of a pain response. What’s curious about the pain placebo is that the effect can occur even when people know they are taking a placebo.
This pain suppression pathway doesn’t just respond to obvious contextual cues and expectations. Even seemingly unrelated cognitive activities can influence pain perception in surprising ways.
Ever wonder why unleashing a torrent of profanity can make your stubbed toe hurt less? There’s a scientific reason behind it!
Research suggests that swearing can help reduce pain intensity. But there’s a catch! This pain-relieving effect of swearing seems only true for people who don’t typically swear. So, save up those curse words; you never know when you might need them.
The Sum Up and Next Week…
While I have divided pain into the painfulness, suffering, and suppression pathways for ease of explanation, in reality, these pathways are not entirely distinct, independent and static — they are interconnected and constantly changing.
I started this article with one paradox of pain — pain is something we want to avoid, but experiencing pain is very important.
There is a second paradox of pain — there doesn’t seem to be a place in the brain where pain happens. There are many brain areas active during pain, but no single pain centre.
So, what exactly is pain?
As some of you have already mentioned, there is one phenomenon that challenges the neuroscience — phantom limb pain.
Imagine feeling intense pain in your left arm, but you don’t actually have a left arm.
This puzzling condition raises questions about the nature of pain. Is pain purely a creation of the brain? Or does our current neuroscientific model fall short?
Next week, we’ll explore the fascinating case of phantom limb pain. We’ll discover a new theory that not only attempts to explain this perplexing phenomenon but also offers an intriguing perspective as to why we feel pain at all.
Thank you.
I want to take a small moment to thank the lovely folks who have reached out to say hello and joined the conversation here on Substack.
If you'd like to do that, too, you can leave a comment, email me, or send me a direct message. I’d love to hear from you. If reaching out is not your thing, I completely understand. Of course, liking the article and subscribing to the newsletter also help the newsletter grow.
If you would like to support my work in more tangible ways, you can do that in two ways:
You can become a paid subscriber
or you can support my coffee addiction through the “buy me a coffee” platform.
I want to personally thank those of you who have decided to financially support my work. Your support means the world to me. It's supporters like you who make my work possible. So thank you.
Fantastic, Suzi. Thank you.
I got very frustrated in my class on philosophy of mind because the tutors always wanted to use pain as an example of why the mind is separate from the brain but they barely had an understanding of how pain works themselves. I know a little bit more now!
I hope you are able to say more about the insula at some point (I have a tumour in mine). My understanding is that it pulls together all the senses to create a big picture view of what is going on around me and some role in emotions that I don't quite understand. Is that close?
An excellent overview Suzi!
The second paradox of pain is something I've always found very interesting, that there is no one thing in the brain, no one circuit or center, where pain happens. The only convergence, based on what I've read, is in our categorization of the particular experience as "pain". That's caused some (Daniel Dennett, Jennifer Corn, etc) to take an eliminative attitude toward it. But pain seems undoubtedly useful as a concept in everyday talk, so I think the right approach is to figure out the mapping between the everyday concept and all the disparate underlying phenomena.
But it's also a stark reminder that a psychological concept, in many cases, won't cleanly map to a neurological one. A psychological kind isn't necessarily a natural kind, or maybe more precisely, isn't just one kind, but could be numerous different kinds.